Hearing light – Demonstrations of Auditory Sensitivity, Part I
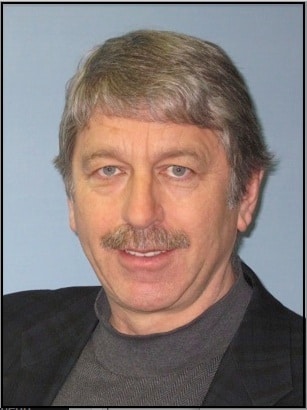
Dr. Manfred Euler
Hearing Health and Technology Matters is pleased to have Dr. Manfred Euler, of the Leibniz-Institute for Science and Mathematics Education (IPN), Kiel, provide the answer to the question, “Can you hear light?” Not only does he answer the question, but he provides simple techniques and kitchen tools to demonstrate this in a two-part series.
Manfred Euler received his Ph.D. in physics from the University of Giessen (Germany). Until his retirement in 2013, he was Director of the Physics Education Department at the Leibniz-Institute for Science and Mathematics Education (IPN) and Professor of Physics Education at the University of Kiel. Before that, he held university positions in Duisburg, Hannover and Paderborn. His research interests comprise various fields in physics and in science education, including research projects on teaching and learning modern physics, especially complex systems, nonlinear dynamics & biophysics. He has run various national and international R&D projects in the area of quality development in physics education and on inquiry-based science teaching and learning.
Wayne Staab, Editor
The remarkable performance of the human auditory system
Our ear is a high-performance sensory organ endowed with an amazing sensitivity. We are able to detect and discriminate sound signals over a wide span of energies and frequencies. Depending on the age of a person, the audible tone spectrum covers up to 10 octaves. Under most favorable circumstances we can discriminate frequencies with a relative precision of 0.3%. Moreover, the auditory system adapts to rapidly varying sound signals within a wide dynamic range. It is able to cope with sound intensities that differ by 12 orders of magnitude from the faintest just audible whisper at hearing threshold (0 dB HL) to the painful roaring thunder of a jet airplane during takeoff (120 dB SPL). The wide span of signal energies is comparable to a sensor capable of reliably detecting the impact of a flea or an elephant. Compared to the workings of vision, auditory mechanisms are much less transparent and more difficult to grasp. In order to demonstrate the amazing performance of hearing we present a series of experiments which can be carried out easily with common kitchen equipment.
Visualizing the sensitivity of hearing
Most people (especially men) will know about the acoustic power installed in their home or car audio systems, typically ranging from tens of Watts up to several hundred Watts. However, they have no idea how these figures relate to the threshold of hearing. Conduct a test and ask your friends and colleagues: “What is the minimum sound intensity in terms of W/cm2 that the human ear can hear?”
The unit W/cm2 was chosen because its numerical value corresponds largely to the energy of a sound wave that passes in one second through the ear canal (cross section area ≈ 1 cm2). People will guess numbers in the milli- to nano-Watt range (10-3-10-9 W). Even those with a background in physics or electrical engineering go wrong by many orders of magnitude. Under most favorable conditions (sound frequency ≈ 3 kHz), a threshold intensity of I0 ≈ 0.5 x 10-16 W/cm2 is audible.
Such tiny quantities are difficult to grasp and require visualization. The threshold is equivalent to detecting a signal source with a power of 10 W at a distance of 1000 km, provided that there is no absorbing medium in between. Imagine, for instance, seeing an energy-saving lamp that far off, a really intriguing performance (Figure 1). The sensitivity of the biological sensor system ear is comparable to high-tech products such as microwave receivers in satellite dishes. In order to figure out the threshold terms of electronic energies, assume a particle gun that accelerates electrons by the potential difference of a standard 1.5 V battery. The impact of 100 electrons per second would become audible.
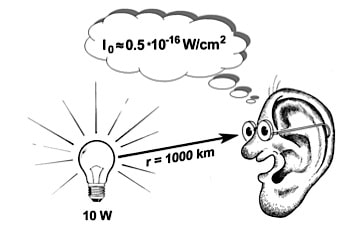
Figure 1. Visualizing the threshold of hearing in terms of the minimum audible intensity I0.
If the ear were a bit more sensitive, we could literally “hear the grass grow.” Decreasing the threshold only a little further would bring us in direct contact with the atomic processes of the world on the nanometer scale. We could detect atoms by their noise, when they bounce irregularly upon the eardrum due to thermal motion. Evolution has driven ear performance to the physical limits. Its sensitivity is bounded from below by thermal noise. Calculating the noise level is a task for experts and requires non-trivial theoretical arguments. Are there more direct ways of demonstrating the sensitivity by simple experiments that will convince the expert as well as the layperson?
Such experiments do exist! Take Figure 1 literally and ask your colleagues and friends if it is possible to hear light. After a short discussion of your mental soundness, engage them in a bet. Wager that the sensitivity of the human ear is sufficient to hear the light emitted by an incandescent bulb. You will win, because you can justify your claim by perceptual experiments. Of course, there is a trick involved that transforms light into an audible signal! The experiments are based on energy conversion chains and include signal amplification via resonance. These processes also play a central role in auditory mechanisms. The idea emerged during a seminar on the biophysics of hearing and was stimulated by discussing Figure 1{{1}}[[1]]Euler, M., Niemann, K. & Müller, A. Hearing Light. The Physics Teacher 38, 356 (2000); doi: 10.1119/1.1321818[[1]].
Designing photoacoustic detectors with kitchen materials
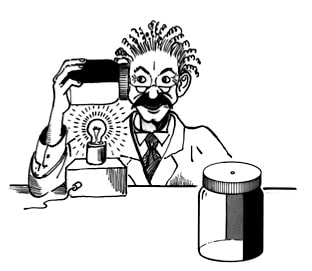
Figure 2. Hearing light with a pickle jar as a photoacoustic converter.
Here is the cookbook recipe to create a system that converts light from incandescent lamps to an audible signal, a so-called photoacoustic detector. Take an empty pickle jar (Volume ≈ 750 ml) and make a circular opening (diameter ≈ 3 mm) in the metal lid. The shape of the glass container (cylindrical or spherical) is not critical. Use a tea-light candle to blacken the glass wall from the inside by precipitating soot from the candle flame. Try to cover half of the inner surface with soot, but leave the opposite glass wall transparent. Close the jar with the perforated lid and hold the hole tight to your ear. Use an AC-powered bulb to shine light on the soot-covered inner surface through the transparent opposite side of the jar (Figure 2). You will hear a low-frequency hum.
High voltage halogen bulbs (60 Watt) will produce excellent results. Energy saving lamps will not work. Definitely, the tone is caused by the incoming light because it will cease after you block the light path by inserting your hand. The experiment is surprising and thought provoking. How is it possible that light can elicit an audible tone? There are three main factors that make the pickle jar experiment work:
(1) Photoacoustic conversion is based on the absorption of light and the transfer of thermal energy to sound waves. This process takes place within the soot-covered layer and the interface between soot and air.
(2) It is necessary that the incoming radiation fluctuate periodically. As the light bulb is powered by AC, the electric energy input oscillates and fluctuations of the light intensity with twice the main frequency will result.
(3) An amplification mechanism is required. The glass container with the sound opening acts as an acoustic resonator. It amplifies the weak photoacoustic signal to an audible level.
The bulb’s hot tungsten filament emits visible and infrared light. The porous soot layer is a perfect absorber. The black body transforms the incoming light almost completely into thermal energy, which is transferred to the boundary layer of the adjacent air by thermal conduction. The air heats up. Thermal expansion creates a pressure pulse inside the jar. To elicit a steady tone, there must be a periodically varying energy input generating a continuous sequence of pressure pulses.
The AC power supply with a frequency of 50 Hz (or 60 Hz, depending on the country) provides the required oscillatory process. The filament current drops to zero twice per period. As a consequence, the filament temperature rises and falls periodically. The emitted light intensity fluctuates in synchrony with each half wave of the AC supply and creates a photoacoustic signal with twice the line frequency. Depending on where the experimenter lives, a tone with 100 Hz or 120 Hz can be heard. The thermal inertia of the filament has a smoothing effect and reduces the light intensity fluctuations. Thus, the resulting sound signal is weak and requires selective amplification to become audible.
Part II of “Hearing Light” will be posted next week with demonstrations of auditory sensitivity.