By Hillary K. Siddons and Frank E. Musiek, University of Connecticut
The cerebellum is a complex neural structure located at the base of the skull in the posterior fossa. It lies caudal to the occipital lobe of the brain and dorsal to the brainstem, at the level of the pons and the medulla. The cerebellum is separated from the brainstem by the fourth ventricle, but is also connected to the brainstem via three peduncles:
- The superior cerebellar peduncle
- The medial cerebellar peduncle
- The inferior cerebellar peduncle
In the classical view, the cerebellum primarily functions as a motor brain region. It is responsible for many functions, including the maintenance of balance, postural control, gait stability, coordination of voluntary movements, accuracy of movements, sensorimotor integration, as well as motor learning (Furman, 2001).
Though the cerebellum is traditionally and primarily viewed as a motor structure, it is important to recognize that the cerebellum is a developmental derivative of the dorsal half of the neural tube, which is the embryological source of sensory structures in humans (Herrup & Kuemerle, 1997). Therefore, the cerebellum is also a major associative center for sensory input integration, including auditory stimuli, which will be the focus of this article.
The cerebellum is also involved in cognitive functions such as attention, language, and emotion. Notably, research has demonstrated that there are, in fact, afferent and efferent neural connections between the cerebellum and both motor and sensory areas of the human brain, including the central auditory nervous system.
Understanding the Anatomy
It is useful when viewing the basic anatomy of the cerebellum to consider its proximity to the brainstem (Figure 1).
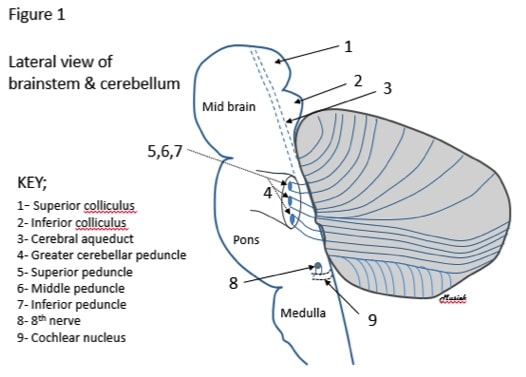
Figure 1. A sketch of a lateral view of the brainstem and cerebellum.
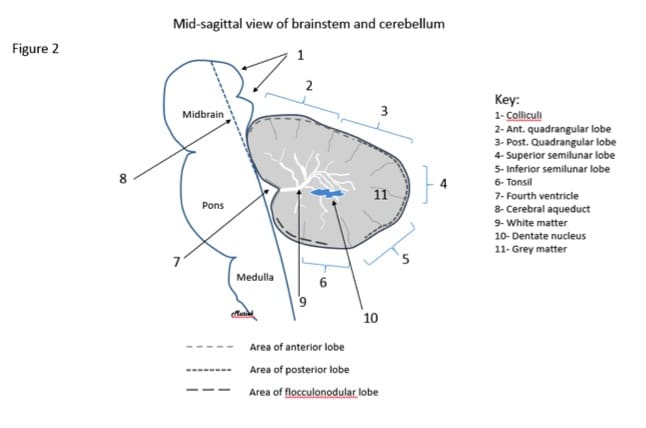
Figure 2. A drawing of a mid saggittal view of the brainstem and cerebellum and the anatomy at this site. Note the three major lobes indicated by various dashed lines and the minor lobes in this area (numbers 2-6).
At the cellular level, the cerebellum is made up of gray matter and white matter (Figure 2). The outermost layer is gray matter, which is made up of cell bodies. The gray matter makes up the cerebellar cortex. The inner portion of the cerebellum consists of white matter, which is made of multiple axons and is responsible for transmitting neural impulses.
Additionally, there are four cerebellar nuclei: the fasitgial nucleus, the globus nucleus, the emboliform nucleus, and the dentate nucleus.
These nuclei are made up of gray matter and lie within the white matter of the cerebellum. Anatomically, the cerebellum can be transversely divided into three major anatomic regions:
- The anterior lobe
- The posterior lobe
- The flocculonodular lobe
Further, Schmahmann and colleagues (2000) discovered that these three anatomical areas can further be divided into ten lobules. The anterior lobe is comprised of lobules I through V. The posterior lobe is made up of lobules VI through IX and the flocculonodular lobe consists of lobule X.
The cerebellum can also be divided, sagitally, into three subdivisions. Specifically, there are two lateral hemispheres that are separated by the most medial region of the cerebellum, called the vermis. Functionally, the cerebellum can be divided into three longitudinal regions based on afferent projections: 1) the vestibulocerebellum; 2) the spinocerebellum; and 3) the pontocerebellum.
The vestibulocerebellum is made up of the flocculonodular lobe and the fastigial nuclei. This region receives afferent impulses from the lateral vestibular nuclei providing the cerebellum with head and body orientation information (Walker, 2010).
The spinocerebellum is made up of the vermis and the fastigial, globose, and emboliform nuclei. It receives and integrates sensory impulses from muscles and tendons. This network is critical for posture control, muscle tone, and coordination of voluntary movements.
The pontocerebellum consists of the lateral hemispheres and the dentate nuclei. It has afferent and efferent connections with the cerebral cortex and the pons via the cerebropontocerebellar tract to provide the cerebellum with information about motor programming. The pontocerebellum also assists with fine tuning in the planning of voluntary motor movements and muscle coordination (Walker, 2010). It also plays a role in cognitive function.
Anatomical Auditory Connections to the Cerebellum
Interestingly, there are evident neural anatomical connections between the central auditory nervous system and the cerebellum. In fact, the discovery of auditory input to cerebellum dates back to the 1940s. Snider and Stowell (1944) conducted the pioneering studies of auditory cerebellar research in which they determined and mapped cerebellar auditory areas by studying the occurrence and distribution of electrical evoked responses.
Specifically in cats, they found the vermis area of the cerebellum receives inputs from auditory, tactile, and visual receptors and transmits information to the cortical pathways (lobules VI and VII).More specifically, there are neuronal projections from the cochlear nucleus, a critical auditory structure, to the vermis area of the cerebellum in cats (Niemer & Cheng, 1949; Huang et al., 1982; Zhang et al., 1990) and to the dentate nuclei in the lateral hemispheres (Wang et al, 1991). Additionally, Sun and colleagues (1983) discovered via microelectrode mapping that the cerebellar vermis and some areas of the lateral hemispheres respond to acoustic stimuli in mustache bats. Figure 3. Illustrates the direct neural connections between the central auditory nervous system and the cerebellum.
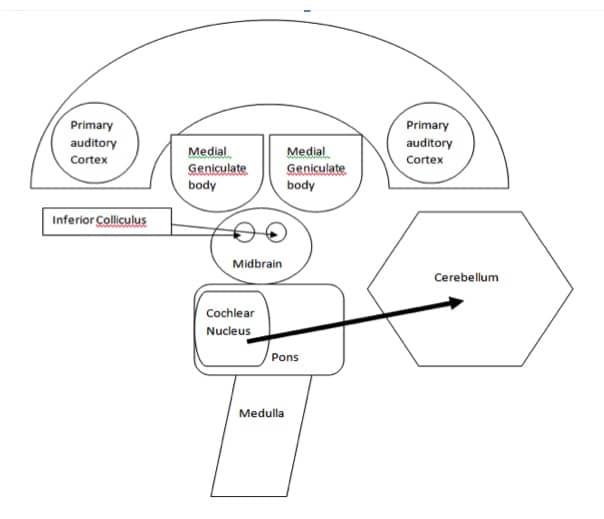
Figure 3. A block diagram of the direct connections between the central auditory nervous system and the cerebellum.
Further, indirect auditory connections to the cerebellum have also been documented in the literature. Specifically, neural fibers arising from the inferior colliculus project to the pontine nuclei and then project to the cerebellar cortex via the middle cerebellar peduncle (Siegel & Sapru, 2010).
The medial geniculate body and the auditory cortex have also been shown to communicate indirectly with the vermis of the cerebellum via the dorsolateral pontine nuclei (Aitkin and Boyd, 1978; Huffman and Henson, 1990). Figure 4. Illustrates the indirect connections between the central auditory nervous system and the cerebellum.
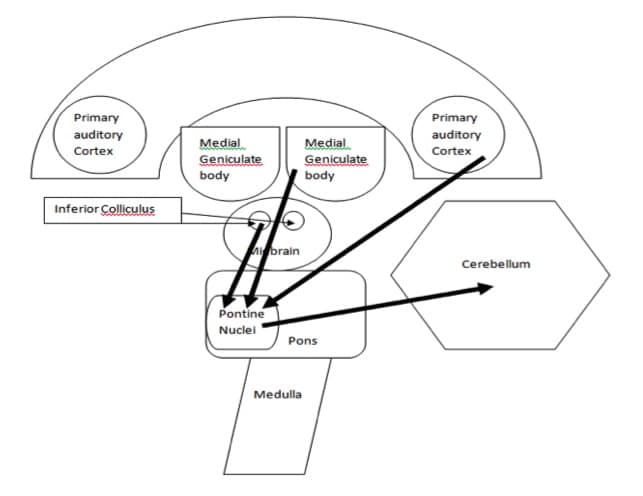
Figure 4. A block diagram of the indirect neural circuitry between the central auditory nervous system and the cerebellum.
Physiological and Functional Evidence for Auditory Functions in Cerebellum
In addition to anatomical studies, there is also evidence from electrophysiological measures and imaging studies suggesting a functional connection between the auditory system and the cerebellum.
Wolfe (1972) demonstrated that the vermis shows frequency specificity and has thresholds as low as those seen in the primary auditory cortex. Also, the vermis is particularly sensitive to changes in intensity and directionality of the sound source. Wolfe’s research supports the notion that the cerebellum is in fact a central auditory structure, in that it responds similarly to other structures along the ascending auditory pathway.
Further, Xi and colleagues (1994) demonstrated that the dentate nucleus of the cat cerebellum responds with short latencies of 4-14 ms to acoustic stimuli as measured by intracellular recordings. This evidence suggests that the cells of the dentate nucleus are a component of a primary ascending auditory transmission pathway between cochlear nuclei and the motor cortex.
Pastor and colleagues (2002) investigated the neurophysiology of normal human brains in response to auditory signals at various frequencies. They used functional brain imaging with positron emission tomography (PET) to demonstrate that 40 Hz selectively activated some areas of the lateral hemispheres, which make up the pontocerebellum. Notably, in the human auditory system, 40 Hz is the stimulation frequency that yields the highest amplitude regarding electroencephalographic (EEG) steady-state responses.
Therefore, these findings suggest the lateral hemispheres, the pontocerebellum, may be a responsive auditory region of the cerebellum. This provides evidence to support the functional role of the cerebellum in the human auditory system.
Secondly in a review of PET studies, Petacchi and colleagues (2011) demonstrated cerebellar involvement during passive listening, specifically in the area of lobule IX. Additionally, activity was elicited in multiple regions of the cerebellum during a pitch discrimination task, namely crus I, crus II, lobule VI, lobule VII, and lobule VIII. Interestingly, increasing the difficulty of the pitch discrimination task yielded increased activity in lobules V, VI, and IX. These findings further support the contribution of the cerebellum in the acquisition of auditory information.
Thirdly, Baumann and Mattingley (2010) investigated the role of the human cerebellum regarding the processing of auditory stimuli, using functional magnetic resonance imaging. fMRI results revealed symmetrical activation of the medial aspect of lobule VI, bilaterally. Results also revealed lateral activation of the right-hemispheric lobules VIII and VIII in response to acoustic stimulation. Further, the left crus I of the cerebellum increased in activation as the difficulty of the task increased, specifically, as the intensity of the target stimulus decreased. The activation of the cerebellum during acoustical stimulation indicates that there are areas of the cerebellum that seem to contribute to the processing of acoustic signals. Additionally, the increase of cerebellar activation as task difficulty increased further supports that the cerebellum contributes to the acquisition of acoustic signals.
Lesions studies also provide insight regarding the role of the cerebellum in auditory processing. Specifically, patients with cerebellar degeneration demonstrate deficits in perceptual timing tasks, suggesting that cerebellar integrity is an important neural structure in terms of temporal processing. This provides further evidence for the role of the cerebellum is not only motor functions, but plays a critical role in sensory processing as well, specifically fine auditory processing (Ivry and Keele,1989; Nichelli et al.,1996; Harrington et al., 2004; Pastor et al.,2004].
Further, Parsons and colleagues (2009) investigated auditory sensory function in patients with global cerebellar degeneration on the performance of a pitch discrimination task. Results demonstrated patients with global atrophy of the cerebellum had significantly poorer pitch discrimination thresholds, despite normal pure-tone thresholds and verbal auditory memory when compared to normal controls. These results indicate that auditory sensory function, specifically sensory acquisition and processing, is affected by cerebellar dysfunction.
Clinical Implications
After reviewing the literature, it is quite evident that the cerebellum has a set of regions that are responsive to auditory stimuli. Additionally, damage to the cerebellum appears to have negative effects in terms of performance on auditory tasks, specifically in terms of temporal and pitch perception. However, the actual role of the cerebellum and the extent of cerebellar involvement in auditory acquisition and auditory processing remain unclear.
In spite of this, considering the responsibilities of the cerebellum in terms of the motor system, one can speculate about the possible functions of the cerebellum regarding the auditory system. Specifically, in the motor system the cerebellar cortex receives and integrates sensory afferent information from the spinal cord, the vestibular labyrinth, the visual system, and the cortex. This integrated signal is then transmitted deeper within the cerebellum to the cerebellar nuclei which further integrate the signals from the cerebellar cortex with direct afferents from the spinal cord, vestibular labyrinth, the visual system, and the cortex. Based on the impulses received, the cerebellar nuclei send efferent signals to the motor system to make smooth, fine motor adjustments and to maintain balance (Walker, 2010).
Overall, the role of the cerebellum in the motor system appears to be twofold. First, it acts as an integration center. Secondly, the cerebellum functions as a fine-tuner. Therefore, the cerebellum may act as a sensory integrator and a fine-tuner in the auditory system as well. Taking into account the deficits on pitch discrimination and perceptual timing tasks discussed earlier in this article, it is important to consider the possible effects that damage to the cerebellum may have on our clinical tests.
Speculating, lesions to the cerebellum probably will not impact basic audiologic testing in terms of the pure-tone audiogram or speech reception/recognition testing, in that these tasks only assess detection and identification auditory capabilities, respectively. However, more demanding tests such as a central auditory test battery are where some influences may come to light. Since cerebellar lesions have been demonstrated to impair perceptual timing tasks, there possibly could be some effects on tests of temporal processing skills, such as temporal ordering or sequencing and temporal resolution. Clinicians should be mindful of individuals with cerebellar deficits, in that, manifestations of this kind of disorder possibly could influence more demanding tests of higher auditory function.
References
- Aitkin, L.M., & Boyd, J. (1978). Acoustic input to the lateral pontine nuclei. Hearing Research, 1, 67–77.
- Baumann, O. & Mattingley, J. B. (2010). Scaling of Neural Responses to Visual and Auditory Motion in the Human Cerebellum. The Journal of Neuroscience, 30(12), 4489-4495. doi: 10.1523/JNEUROSCI.5661-09.2010
- Furman. J. (2001). Performing the Physical Examination: Cerebellar Tests. In J. Goebel (Ed.), Practical management of the dizzy patient (pp. 99-108). Lippincott Williams & Wilkins.
- Harrington, D.L., Lee, R.R., Boyd, L.A., Rapcsak, S.Z., Knight, R.T. (2004). Does the representation of time depend on the cerebellum: effect of cerebellar stroke. Brain, 127, 561-574.
- Herrup, K., & Kuemerle, B. (1997). The compartmentalization of the cerebellum. Annual Review of Neuroscience, 20, 61–90.
- Huang, C. M., Liu, G., & Huang, R. (1982). Projections from the cochlear nucleus to the cerebellum. Brain Research, 244, 1– 8.
- Huffman, R.F., & Henson, O.W. Jr. (1990). The descending auditory pathway and acousticomotor systems: connections with the inferior colliculus. Brain Research Review, 15, 295–323.
- Ivry, R.B., Keele, S.W. (1989). Timing functions of the cerebellum. Journal of Cognitive Neuroscience, 1, 136-152.
- Nichelli, P., Alway, D. & Grafman, J. (1996) Perceptual timing in cerebellar degeneration. Neuropsychologia, 34, 863-871.
- Niemer, W.T., Cheng, S. (1949). The ascending auditory system, a study of retrogradedegeneration. Anatomy Research, 103, 116.
- O’Reilly, J., Beckmann, C. F., Tomassini, V., Ramnani, N., & Johansen-Berg, H. (2010). Distinct and Overlapping Functional Zones in the Cerebellum Defined by Resting State Functional Connectivity.
- Parsons, L.M., Petacchi, A., Schmahmann, J.D., Bower, J.M. (2009). Pitch discrimination in cerebellar
- Pastor, M.A., Day, B.L., Macaluso, E., Friston, K.J., & Frackowiak, R.S. (2004). The functional neuroanatomy of temporal discrimination. Journal of Neuroscience, 24, 2585–2591.
- Pastor, M.A., Vidaurre, C., Fernández-Seara, M.A., Villanueva, A., & Friston, K.J. (2008). Frequency-specific coupling in the cortico-cerebellar auditory system. Journal of Neurophysiology, 100, 1699-1705.
- Petacchi, A., Laird, A.E., Fox, P.T., & Bower, J.M., 2005. Cerebellum and auditory function: an ALE meta-analysis of functional neuroimaging studies. Human Brain Mapping, 25, 118–128.
- Petacchi, A. Kaernbach, C., Ratnam, R., & Bower, J.M. (2011). Increased activation of the human cerebellum during pitch discrimination: A positron emission tomography (PET) study. Hearing Research, 282, 35-48.
- Schmahmann, J., Doyon, J., Toga, A., Petrides, M., & Evans, A. MRI Atlas of the Human Cerebellum. San Diego, CA: 2000.
- Siegel, A., and Sapru, H. N. (2010). Essential Neuroscience. Philadelphia: Lippincott Williams & Wilkins.
- Snider, R.S., & Stowell, A. (1944). Receiving areas of the tactile auditory, and visual systems in the cerebellum. Journal of Neurophysiology, 7, 331-358.
- Sun, X., Jen, P.H., & Kamada, T. (1983). Mapping of the auditory area in cerebellar vermis and hemispheres of the mustache bat. Brain Research, 271, 162–165.
- Walker, H. K (2010). The Cerebellum. In Walker, H. K., Hall, W. D., & Hurst, J. W., (Eds.), Clinical Methods: The History, Physical, and Laboratory Examinations. Boston: Butterworths.
- Wang, X.F., Woody, C.D., Chizhevsky, V., Gruen, E., & Landeira-Fernande, J. (1991). The dentate nucleus is a short-latency relay of a primary auditory transmissionpathway. Neuroreport, 2, 361–364.
- Wolfe, J.W., 1972. Responses of the cerebellar auditory area to pure tone stimuli. Explore Neurology, 36, 295-309.
- Xi, M.C., Woody, C.D., & Gruen, E. (1994). Identification of short latency auditory responsive neurons in the cat dentate nucleus. Neuroreport, 5, 1567–1570.
- Zhang, S., Sun, X., & Jen, P.H.S. (1990). HRP study of neural projections of acoustic signal to the cerebellum of echolocating bats. Acta Theriol. Sin. 10, 110–113.
**this piece has been edited for clarity. It originally published on August 6, 2014
Nice article! There really is a need for a proper review of the role of the cerebellum (and the vermis in particular) in auditory processing, I feel. Having done so much of the groundwork already, do you perhaps have any plans to turn this into a full-fledged peer-reviewed review paper?
Aside, concerning the clinical relevance that you seem to be interested in, an increasing number of studies also implicate the cerebellum in the mechanism behind tinnitus, you may be interested to hear*.
Congrats with the impressive work!
DL
* some example papers linking tinnitus to the cerebellum:
Boyen, K., de Kleine, E., van Dijk, P., Langers, D.R.M., 2014. Tinnitus-related dissociation between cortical and subcortical neural activity in humans with mild to moderate sensorineural hearing loss. Hear. Res. 312, 48–59. doi:10.1016/j.heares.2014.03.001
Boyen, K., Langers, D.R.M., de Kleine, E., van Dijk, P., 2013. Gray matter in the brain: differences associated with tinnitus and hearing loss. Hear. Res. 295, 67–78. doi:10.1016/j.heares.2012.02.010
Lanting, C.P., de Kleine, E., Eppinga, R.N., van Dijk, P., 2010. Neural correlates of human somatosensory integration in tinnitus. Hear. Res 267, 78–88. doi:10.1016/j.heares.2010.04.006
Lanting, C.P., de Kleine, E., van Dijk, P., 2009. Neural activity underlying tinnitus generation: results from PET and fMRI. Hear. Res 255, 1–13. doi:10.1016/j.heares.2009.06.009
Maudoux, A., Lefebvre, P., Cabay, J.-E., Demertzi, A., Vanhaudenhuyse, A., Laureys, S., Soddu, A., 2012. Auditory resting-state network connectivity in tinnitus: a functional MRI study. PLoS ONE 7, e36222. doi:10.1371/journal.pone.0036222
Osaki, Y., Nishimura, H., Takasawa, M., Imaizumi, M., Kawashima, T., Iwaki, T., Oku, N., Hashikawa, K., Doi, K., Nishimura, T., Hatazawa, J., Kubo, T., 2005. Neural mechanism of residual inhibition of tinnitus in cochlear implant users. Neuroreport 16, 1625–1628.
Well written and formulated. Thank you. A totally new way of appreciating the cerebellum. Can you comment on the possibility of the role of the cerebellum in the ability to localise to sound.
Really interesting article. Perhaps a new line of investigation could use evidences of abnormal tests results in patients with cerebellar lesions, specifically vermis tumours (for example retrocochlear findings in ABR)
This is nice information to share with us thanks.
https://blog.mindvalley.com/cerebrum-vs-cerebellum/