Ear Canal Length
Most ear canal dimensional measurements relate to the length of the ear canal. However, measurements of the ear canal length are not straightforward because measurement methods vary, especially as to how the distal and proximal boundaries of the ear canal are identified. Additionally, ear canal length provides no information about the ear canal configuration, which has been an even more difficult, but more important, measurement to obtain.
Perhaps some of the 3D imaging currently being used to make ear impressions will gather data into a database to enhance this knowledge, but this has not happened yet. By far the most used method for gathering such data involves physical measurements of ear impressions made on either live persons or on cadavers. This post is a continuation of last week’s post on the human ear canal.
Measurement procedures that have been used to determine ear canal length include the following:
Physical Measurements
- Direct physical measurements of ear canal impressions. These have the advantage of providing information about the configuration as well as the dimensions of the canal. The difficulty with this method is that making impressions of the complete ear canal, including the imprint of the tympanic membrane, is no simple task. As a result, most physical measurements are made from ear impressions of cadaver ears. The primary question related to cadaver ear measurements is whether they continue to represent living ear canals. Thousands of complete ear impressions were made on live individuals for the Philips XP Peritympanic hearing aid in the mid-1990s. Unfortunately, most of the actual ear impressions were destroyed, but some of the data gathered (by this author) is presented in this series of posts.
The length of the ear canal is determined by the identification of the proximal and distal boundaries of the ear canal. These specific points are seldom, if ever, identified in reported measurements, making it difficult to compare results. An advantage of physical measurements of the ear impression is that they can provide information as to the overall dimensions and shape of the ear canal.
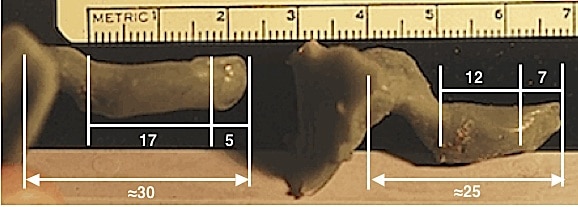
Figure 13. Ear impressions of the complete external auditory canal, including imprints of the tympanic membrane, taken from live subjects. (Wayne Staab photos).
The posteriosuperior wall of the external canal measures approximately 25 mm in length to the TM (tympanic membrane), whereas the anterior-inferior distance to the TM is about 6 mm longer. This 6 mm is the approximate length of the angled tympanic membrane and can be visualized in the ear impression of the complete ear canal, including the TM (Figure 13). The angle of the TM in the adult has been reported to be as great as 60 degrees, whereas in an infant, it could be almost horizontal to the ear canal.
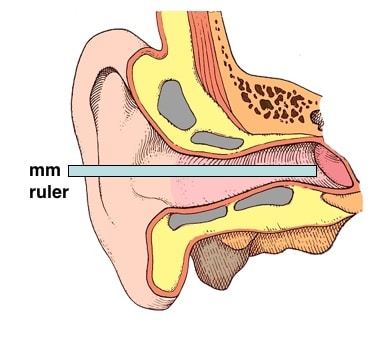
Figure 14. Direct physical measurement of the ear canal length using a measurement gauge inserted into the ear canal until it contacts the tympanic membrane.
The tympanic membrane contributes approximately 6 mm to the overall length of the ear canal, and, as a result, affects the measurement length, depending on the contact area to where the measurement is made. If the measurement is made along a superior-posterior axis, the length will be shorter than if the measurement is made along an inferior-anterior axis. This fact contributes to variations in ear canal length measurements.
2. Inserting a measurement gauge into the ear canal (Figure 14) until it touches the tympanic membrane provides information as to the length, but tells us nothing about the configuration of the ear canal.
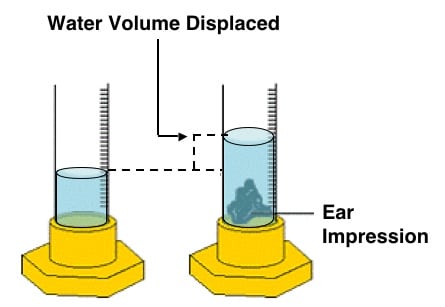
Figure 15. Water displacement method of determining volume, ala Archimedes.no information as to the configuration of the ear canal.
- Another physical measurement (of volume, but not of configuration) has involved the use of a calibrated container filled with a liquid solution (water or a 60% alcohol solution). The volume is a function of the liquid displacement when the ear impression is inserted into the container’s solution (Figure 15).
Optical Measurements
A non-invasive procedure that has been used to measure the length of the ear canal uses a microscope (Figure 16). The microscope is focused on the tympanic membrane. It is then refocused on an earplug in the ear that terminates at the aperture of the ear canal. The focus measurement difference provides information about the length.
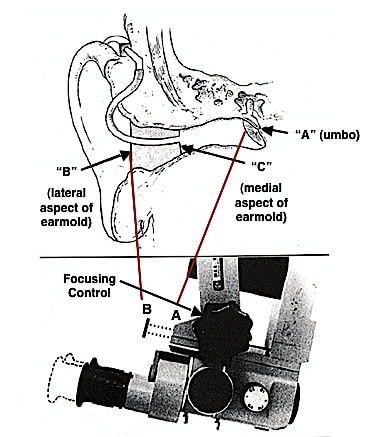
Figure 16. Ear canal length determination using a microscope two-point focal procedure used by Zemplenyi, Gilman, and Dirks, 1985. This method is limited to measurements of length only.
Radiological Measurements
These non-invasive procedures involve CT and MRI scans. The CT is best for identification of bony structure, whereas the MRI is used best for measurement of soft tissues. The primary problems associated with these methods are: (a) differentiating the air/flesh interface, (b) exposure to radiation, and (c) ±5 mm variation in accuracy (at least at the time). Of course, the greatest objection to the use of these techniques is the exposure to radiation.
Figure 17 illustrates a CT scan procedure from the inferior to superior direction to obtain transverse plane measurements of a cadaver head. A comparison of measurements obtained using this method to physical measurements of an ear impression made on the same cadaver head showed that the measurement deviations ranged from 0.18 to 25.1% with a mean value of 9.65%. Volume differences were 6.12%.
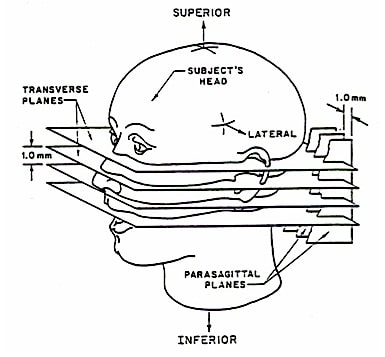
Figure 17. CT scans to determine the configuration and volume of the external auditory canal (Egolf, et. al., 1993).
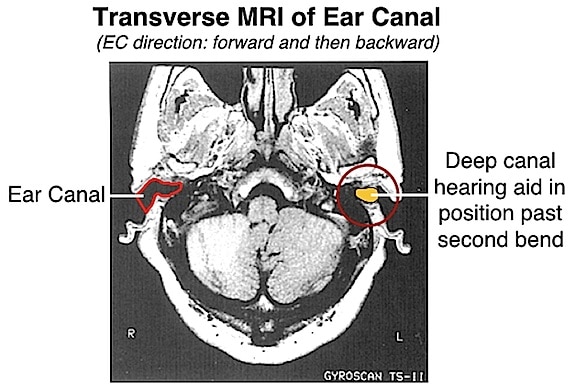
Figure 18. MRI showing configuration of ear canal (left side), and a deep fitting canal hearing aid inserted beyond the second bend (right side).
An MRI of the ear canal section of a live human is shown in Figure 18, along with showing an XP Peritympanic deep canal hearing aid inserted into the left ear
Acoustic Measurement Method (mathematical description using sound pressure levels)
Acoustic procedures generally measure the sound pressure at 2+ locations in the ear canal to obtain the functional area. In one such measurement procedure, Huddle considered the ear canal to be a conduit having varying cross section, and treated it as a lossless acoustic line. Exciting this duct with a broadband sound source (i.e., harmonic signal) would generate a pressure distribution, and from these pressure distribution values, the area of the ear canal could be solved using a linear system of equations and executing a simple iterative algorithm. The linear formula used provided a good approximation of the ear canal, but did not take into consideration the importance of curvature of the canal in calculating the geometry. Later work stated that it has been proved that the measurement of the acoustic pressure on the ear membrane allows one to determine the shape of the ear canal uniquely.
Another less complicated approach to measuring ear canal length is to measure sound pressure levels to a broad band signal at different locations in the ear canal, and determine the maxima and minima at different frequencies. This procedure uses 1/4 wave theory, recognizing that the minima at any frequency occur at a distance of 1/4 of the wavelength of the signal from the TM (i.e., standing waves). These pressure minima are seen as notches in the spectrum from the pressure measurements made. The distance from the TM (length at that location) can be estimated based on the quarter wavelength that corresponds to the frequency, using the formula:
Immittance Measurements
Commercially-available immittance instruments are able to measure the air column between the probe and the tympanic membrane. This is not to be confused with total ear canal volume normally measured with such instruments. An attempt to determine the relationship between the tympanometric estimate and the measured volume suggested that the tympanometric estimate was 33% higher than the actual volume. Shanks and Lilly reported that the tympanometric procedures overestimated the actual physical volume (obtained by filling in space between probe and the TM with alcohol) by 10 to 39% depending on the probe tone frequency.
Reference:
- Staab, W. Philips XP Training Sessions. (1995-1996). Fifty plus training sessions in the U.S. and Internationally
- Perry, E.T. (1957). The human ear canal, Springfield, IL, Charles C. Thomas
- Morton, J.Y., and Jones, R.A. (1956). The acoustical impedance presented by some human ears to hearing and earphones of insert type. Acustica, 6, 339-345
- Zwislocki, J.J. (1971). An acoustic coupler for earphone calibration. Special Report LSC-S-9. Laboratory of Sensory Communication. Syracuse University, New York
- Zemplenyi, J., Gilman, S., and Dirks, D. (1985). Optical method for measurement of ear canal length. JASA, 78, 2146-2148
- Egolf, D.P., Nelson, D.K., Howell, H.C., III, and Larson, V.D. (1993). Quantifying ear-canal geometry with multiple computer-assisted tomographic scans. JASA, 93, 2809-2819
- Staab, W. (1995). Deep canal hearing aids, Chapter 8 in The Human Ear Canal, Bopanna, B. (Ed.), p. 164, Singular, San Diego
- Huddle, H. (1983). Estimation of the area function of human ear canal by sound pressure measurements, JASA, 73, 24-31
- Bopanna, B.B. (1995). Ear canal acoustics, in The Human Ear Canal, Bopanna (Ed.), Singular, San Diego
- Ramm, A.G., The shape of the ear canal. (2005). arXiv:math.AP/0511359v1 14Nov2005
- Chan, J.C.K., & Giesler, C.D. (1990). Estimation of eardrum acoustic pressure and of ear canal length from remote points in the canal. JASA, 87(3), 1237-1247
- Rabinowitz, W. On the input acoustic admittance of the human middle ear. (1977). Unpublished doctoral dissertation. Massachusetts Institute of Technology, Cambridge
- Shanks, J.E. and Lilly, D. (1981). An evaluation of tympanometric estimates of ear canal volume. J. of Speech and Hearing Research, 24, 557-566
Wayne Staab, PhD, is an internationally recognized authority in hearing aids. As President of Dr. Wayne J. Staab and Associates, he is engaged in consulting, research, development, manufacturing, education, and marketing projects related to hearing. His professional career has included University teaching, hearing clinic work, hearing aid company management and sales, and extensive work with engineering in developing and bringing new technology and products to the discipline of hearing. This varied background allows him to couple manufacturing and business with the science of acoustics to bring innovative developments and insights to our discipline. Dr. Staab has authored numerous books, chapters, and articles related to hearing aids and their fitting, and is an internationally-requested presenter. He is a past President and past Executive Director of the American Auditory Society and a retired Fellow of the International Collegium of Rehabilitative Audiology.
**this piece has been updated for clarity. It originally published on June 23, 2014