Basic Principles for Hearing Aid Prosthetic Purposes
Otometric principles, for hearing aid prosthetic purposes, deal primarily with the physics and acoustics of the hearing mechanism, requirements to identify otometry as a scientific approach to hearing aid selection.
Last week’s post reviewed the differences between audiometry and otometry, setting the stage for this week’s further differentiation.
1. Prosthetic requirements are concerned with sound pressure sensing abilities that remain, not those that have been lost (the latter being audiometric threshold testing and identified as Hearing Loss or Hearing Level in dB). Stated another way, so as not to be misunderstood, a hearing instrument applies sound pressure to hearing that remains, not to that which is lost! So, otometry focuses on identifying and using remaining hearing information (suprathreshold information), rather than concentrating on that which was lost, and described by hearing sensitivity, or threshold. As a result, it is necessary to consider residual hearing range in absolute (sound pressure) rather than relative (Hearing Loss) decibels.
The difference is illustrated in Figure 3 (Figures are numbered as a continuation from last week’s post). The deficient ear usually shows abnormally high threshold pressures (MAP) while maximum tolerable pressures (MTP) may show little change from normal, if any. Most hearing aid fitting formulae are based on applying correction values to audiometric thresholds, levels at which normal listening does not occur. Otometry is based on identifying the most comfortable listening sound pressure level (MCLP) within the residual hearing range, the level at which individuals listen, and applying hearing aid sound pressures to that level (Figure 4). The comfort range is only about 6-dB for normal hearing, and this range usually narrows with increasing hearing loss.
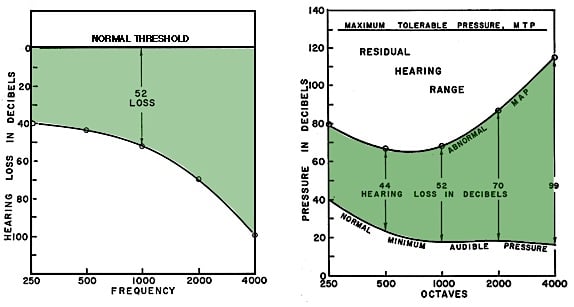
Figure 3. The audiogram (left) and hearing aid fittings based on it, calculate desired amplification from the level of the hearing loss (a relative value). Otometry (right) bases amplification on sound pressure MCL (most comfortable loudness), an absolute value, which lies, when measured, about half way between the recorded abnormal MAP and the maximum tolerable pressure (MTP). The comparisons are: normal threshold (Hearing Loss) compared to normal minimum audible pressure (MAP); and hearing loss in relative dB compared to abnormal MAP in absolute sound pressure.
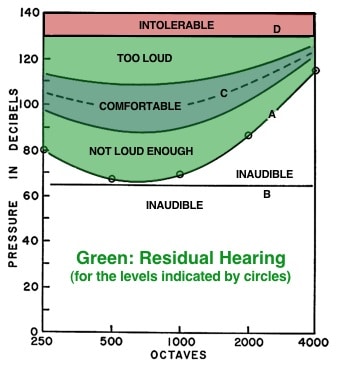
Figure 4. Otometric chart showing a residual hearing range having threshold pressures A, all of which are above the normally received level of line B. Pressures must be raised artificially with a hearing instrument to line C to be most comfortable. Solid lines to either side of dashed line C define a latitude of comfortable loudness pressures.
2. Otometric principles predict what is required to provide maximum utility of residual hearing and do not indicate how much, if any, intelligibility would result. The rationale for this is that prosthetically, it makes no difference whether subnormal hearing is neurological or mechanical in nature.
3. Residual hearing and hearing aid properties must be prescribed in terms of sound pressures required and sound pressures delivered. This means that conventional pure-tone threshold “Hearing Loss” values have to be converted into real minimum audible sound pressures for otometric purposes. However, even if done, minimum audible sound pressures are NOT part of the fitting prescription. They merely show the lower limit of the residual hearing range, which can be useful, along with
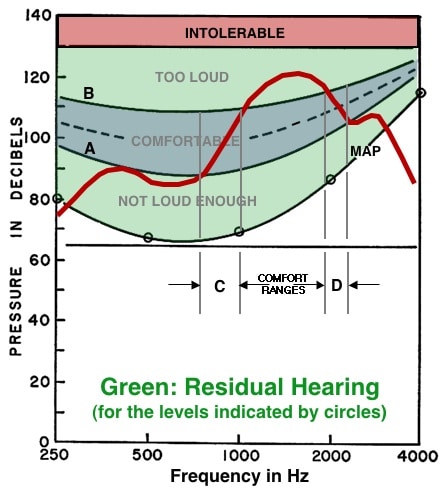
Figure 5. Otometric chart showing how the relative deliverable pressures (RDP) of a hearing instrument (red line) can be superimposed upon the chart of an individual hearing range. The response of the hearing instrument in this example shows that it can provide comfortable listening pressures in only two restricted regions, C and D, instead of distributing the pressures within the comfortable range between A and B. The comfortable level is for illustrative purposes only and does not reflect the most comfortable level pressures for a given individual.
the maximum tolerable pressures, to define the dynamic range from which a comfortable loudness pressure can be interpolated if/when the most comfortable listening pressures are not measured directly (an estimate of mid-point of the residual range). To this effect, audiogram charts are not suitable for prescription purposes, and otometric type charts are used for evaluating residual hearing. In otometry, otometric charts (otograms) and the hearing instrument charts were designed with the same grids so that the measured hearing instrument response could be superimposed on the chart of a residual hearing range to visualize how closely the hearing instrument comes to providing appropriate pressures to meet the comfortable loudness recommendation (Figure 5). The red line represents the measured hearing aid response compared to the comfortable listening level (blue area). In the example shown, the fitted hearing instrument provides comfortable listening in just two restricted regions, C and D. Adjustments would be required to provide as much of the measured hearing aid response (in sound pressure), to fall within the blue shaded area, as possible.
4. Hearing aids/instruments are made to deliver sound pressure. As such, otometric measurement instruments must be more accurate than audiometric instruments if the possibilities of prescription are to be fully realized. In audiometry, thresholds are determined in 5-dB increments, meaning that at any frequency the result could be off by 10 dB because of ±5-dB variability. Pure tones are not acceptable stimuli to make the necessary loudness judgments because of fatigue when louder judgments are to be made, standing waves, etc., resulting in accuracy limitations.
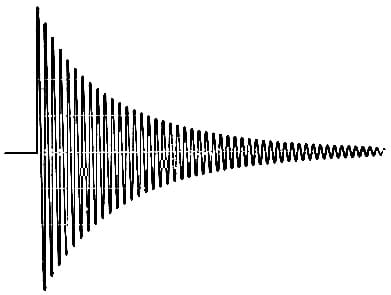
Figure 6. Oscillogram of the exponentially decaying (or damped) oscillatory signal. Each succeeding cycle reduces to 90% of the amplitude of the preceding cycle.
Signal Used – A damped wavetrain (DWT) signal (Figure 6) was designed for use that is more like those produced by the parts of words comprising natural speech{{1}}[[1]]Victoreen, J.A. (1973). Basic Principles of Otometry, Charles C. Thomas, Springfield[[1]]. This signal was selected for the following reasons:
• It is less prone to fatigue
• Signal length should be no longer than necessary for recognition. Obviously, signal duration will vary with the number of cycles per second being delivered. Therefore, delivering the optimum number of cycles in the signal determines signal length. It was determined that about 6 cycles per signal is sufficient for the signal to be recognized. This suggests that for the same purposes, signals should be used that have the same number of cycles per signal, not the same time duration per signal as the frequency is changed. (Loudness sensation changes with the number of cycles that are presented, and also for signals of any constant duration length (Figure 7). This holds true for all signals, including pure tones, narrow band noise, warble tones, etc.).
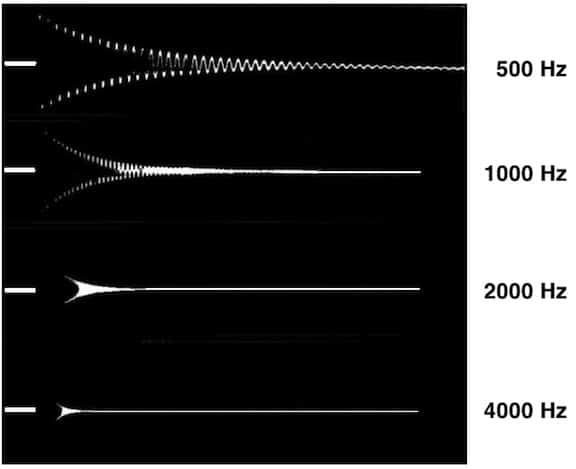
Figure 7. Electric amplitude time appearance of Victoreen decaying (or damped) wavetrain signals for frequencies from 500 to 4000 Hz (Lindström, et. al., 1983). The graph shows that time is not the same for each of the specified frequencies, which it should not be. Instead, the critical feature is the number of cycles.
• Speech and other complex signals contain many individual frequency components, and therefore cannot supply information on a discrete or single frequency basis (needed for most comfortable pressures across the frequency range).
• A wavetrain signal is suitable for measuring purposes because it has a repeatable number of cycles. As a decaying wavetrain signal (damped wavetrain), the decay rate is not specified in time, but in percent of amplitude retained in each succeeding cycle (Figures 6 and 7). A loss of 10% per cycle indicates that 90% remains, so that the decrement is then specified as being equal to 0.90. The signal decreases to about 1/2 amplitude in 6 cycles. The spectral distribution is constant throughout the signal presentation{{2}}[[2]]Lindström, B., Svärd, I., Bredberg, G., and Lundborg, T. (1983). Scandinavian Audiology Supplementum 18[[2]].
5. Free-field (or sound-field) measurements are recommended because both the normal ear, as well as an aided ear, operate in the sound field.
6. Otometry was designed for a closed earmold coupler fitting. Substantial modifications would have to be made for other types of earmold coupling.
7. An adjustable volume control on the hearing aid is important for an otologic prescription fitting.
Next week’s post will take a step-by-step journey of hearing aid selection using otometry.